Seeing More and Brighter Colors with Tyramide Signal Amplification
Propelled by advances in microscopy and the advent of spatial proteomics, seeing more of the molecular orchestra at work in cells and tissues has revolutionized our understanding of biology. With fluorescence microscopy, we can see and measure multiple targets in the cell: proteins in tissues by immunohistochemistry (IHC) and immunofluorescence (IF), and nucleic acids by fluorescence in situ hybridization (FISH). Modern advances in fluorescent dyes, detection, and spectral unmixing technology allow us to image more probes than ever, which is crucial in understanding complex biological tissues (Stack et al., 2014)—multiplexed IHC has shown itself useful in tumor analysis and predicting therapeutic responses to specific cancers (Halse et al., 2018).
Detecting proteins in tissues and cells is commonly done by direct or indirect immunofluorescence, where primary antibodies are either directly labeled or amplified by a fluorescently labeled secondary antibody. However, for low abundance proteins of interest, these techniques may not produce enough light to distinguish specific signal from background autofluorescence. Multiplexing by indirect immunofluorescence is also limited by the source of primary antibodies: cross-reactivity occurs when two primary antibodies are from the same species, so it is typically limited to 3 or 4 colors at best.
What is Tyramide Signal Amplification?
Tyramide signal amplification (TSA), also known as catalyzed reporter deposition (CARD) provides a highly sensitive alternative to IF (Bobrow et al., 1989; Stack et al., 2014). Rather than using antibodies labeled with fluorescent dyes, TSA uses antibodies carrying horseradish peroxidase (HRP), which catalyzes the activation of dye tyramides, which then covalently attach to nearby tyrosine residues (Bobrow et al., 1989).
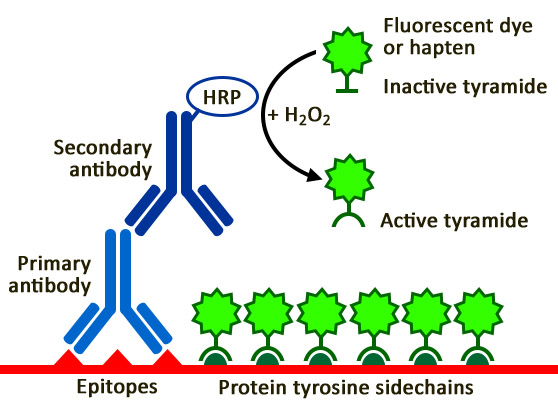
TSA produces a local sea of covalently bound fluorophores near the HRP-conjugated antibody rather than dyes attached to the antibodies themselves. This leaves a permanent fluorescent label in the sample independent of continued antibody binding, allowing more colors to be added by sequential labeling. Thermal or chemical treatment (typically using a microwave and a stripping buffer) removes the primary and secondary antibodies from the sample, and staining can proceed again using a new dye tyramide in a different color. Opal technology (Perkin-Elmer and Akoya Biosciences) pioneered the TSA staining process using dye tyramides and ready-to-use staining and stripping solutions. The bright, photostable, and water-soluble CF® dye tyramides are available in a large range of colors and are an excellent choice for this workflow.
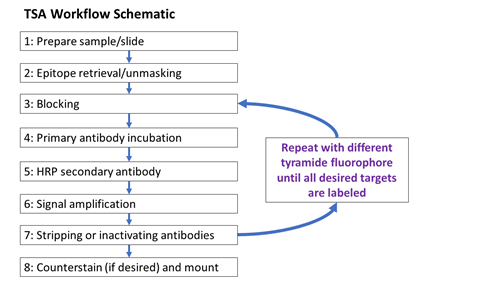
Why use TSA?
TSA offers brighter fluorescent signal than IF and is not limited by antibody cross-reactivity. Like another HRP-based IHC method—the chromogenic 3,3′-Diaminobenzidine (DAB) stain—longer incubation of dye tyramides increases the label density, improving signal brightness. Staining single targets produces similar labeling to DAB, but with fluorescent rather than chromogenic detection (Zhang et al., 2017).
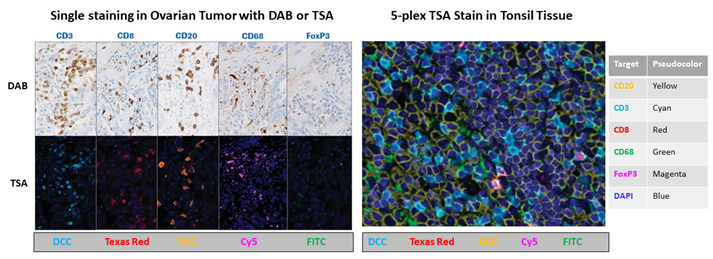
TSA allows higher order multiplexing than immunofluorescence; sequential staining and stripping of antibodies means cross-reactivity is no longer an issue and multiple primary antibodies from the same species can be used—an impossibility with indirect immunofluorescence. There are 20 CF®-tyramide dyes spanning the color spectrum from 350-750 nm; automated slide imagers, like the Orion™ from RareCyte®, or spectral imaging microscopes can render over 20 fluorescence channels in parallel.
With more colors comes a cost: TSA staining of more than 3 colors can be laborious and time-consuming. Using primary antibodies conjugated with HRP can speed up the process but may affect antibody performance and increase costs. Automated slide staining machines such as the Leica® BOND™ are compatible with TSA and perform high-throughput slide staining; this is especially useful in diagnostic or clinical work where molecular profiling of tumors guides personalized medicine or in research settings with a high volume of routine staining.
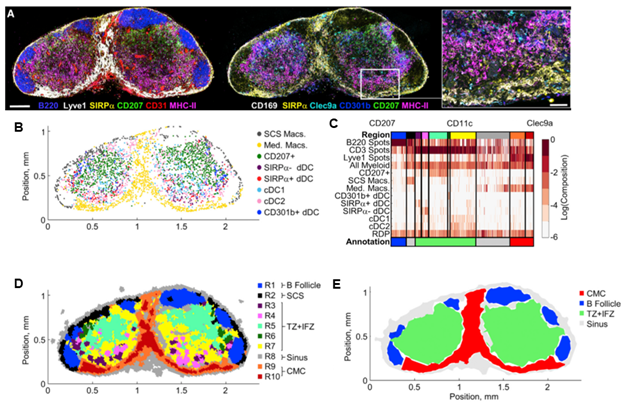
Emerging Applications
TSA is a powerful tool in research and diagnostics. This method can label more simultaneous targets than indirect IF and can be automated, driving deeper understanding of complex tissues—a key to understanding health and disease and advancing patient care (Stack et al., 2014). With the advent of automated tools to map organ cytoarchitecture using high-dimensional staining with CF® dyes, being able to label more than four colors is more advantageous than ever! Lymph nodes (LNs) labeled with 12 colors allowed automated analysis of cellular organization in this complex tissue, revealing that distinct subsets of dendritic cells (DCs) reside in non-overlapping patterns (Stoltzfus et al., 2020).
TSA offers a flexible and cost-effective path to spatial proteomics, producing bright and high-resolution images. For particularly difficult to visualize targets, TSA can be combined with avidin-biotin complex (ABC) staining for further signal amplification; ABC staining with TSA enhances FISH where increased sensitivity or multiplexing is desired, finding such uses in microbial rRNA identification (Amann & Fuchs, 2008). Finally, immunofluorescence can be used after TSA to expand imaging bandwidth.
References
- Amann, R., & Fuchs, B. M. (2008). Single-cell identification in microbial communities by improved fluorescence in situ hybridization techniques. Nature Reviews. Microbiology, 6(5), 339–348. https://doi.org/10.1038/nrmicro1888
- Bobrow, M. N., Harris, T. D., Shaughnessy, K. J., & Litt, G. J. (1989). Catalyzed reporter deposition, a novel method of signal amplification application to immunoassays. Journal of Immunological Methods, 125(1–2), 279–285. https://doi.org/10.1016/0022-1759(89)90104-X
- Halse, H., Colebatch, A. J., Petrone, P., Henderson, M. A., Mills, J. K., Snow, H., Westwood, J. A., Sandhu, S., Raleigh, J. M., Behren, A., Cebon, J., Darcy, P. K., Kershaw, M. H., McArthur, G. A., Gyorki, D. E., & Neeson, P. J. (2018). Multiplex immunohistochemistry accurately defines the immune context of metastatic melanoma. Scientific Reports, 8(1), 11158. https://doi.org/10.1038/s41598-018-28944-3
- Stack, E. C., Wang, C., Roman, K. A., & Hoyt, C. C. (2014). Multiplexed immunohistochemistry, imaging, and quantitation: A review, with an assessment of Tyramide signal amplification, multispectral imaging and multiplex analysis. Methods, 70(1), 46–58. https://doi.org/10.1016/j.ymeth.2014.08.016
- Stoltzfus, C. R., Filipek, J., Gern, B. H., Olin, B. E., Leal, J. M., Wu, Y., Lyons-Cohen, M. R., Huang, J. Y., Paz-Stoltzfus, C. L., Plumlee, C. R., Pöschinger, T., Urdahl, K. B., Perro, M., & Gerner, M. Y. (2020). CytoMAP: A Spatial Analysis Toolbox Reveals Features of Myeloid Cell Organization in Lymphoid Tissues. Cell Reports, 31(3), 107523. https://doi.org/10.1016/j.celrep.2020.107523
- Zhang, W., Hubbard, A., Jones, T., Racolta, A., Bhaumik, S., Cummins, N., Zhang, L., Garsha, K., Ventura, F., Lefever, M. R., Lu, Z., Hurley, J. K., Day, W. A., Pestic-Dragovich, L., Morrison, L. E., & Tang, L. (2017). Fully automated 5-plex fluorescent immunohistochemistry with tyramide signal amplification and same species antibodies. Laboratory Investigation, 97(7), 873–885. https://doi.org/10.1038/labinvest.2017.37