There are reportedly more than a billion tissue samples archived in hospitals and tissue banks around the world, representing a wealth of rich clinical data.1 Most of these samples are formalin-fixed and paraffin-embedded (FFPE). This preservation technique, developed over 100 years ago, remains the gold standard for diagnostics in pathology. FFPE tissue samples are regularly used to diagnose and study disease processes in clinical settings and FFPE processing is common practice for tissue preservation in many non-clinical research laboratories.2 FFPE processing is advantageous for many reasons: it allows for the preservation of morphology, provides convenient long-term storage and sectioning, and reduces the risks associated with infectious agents.
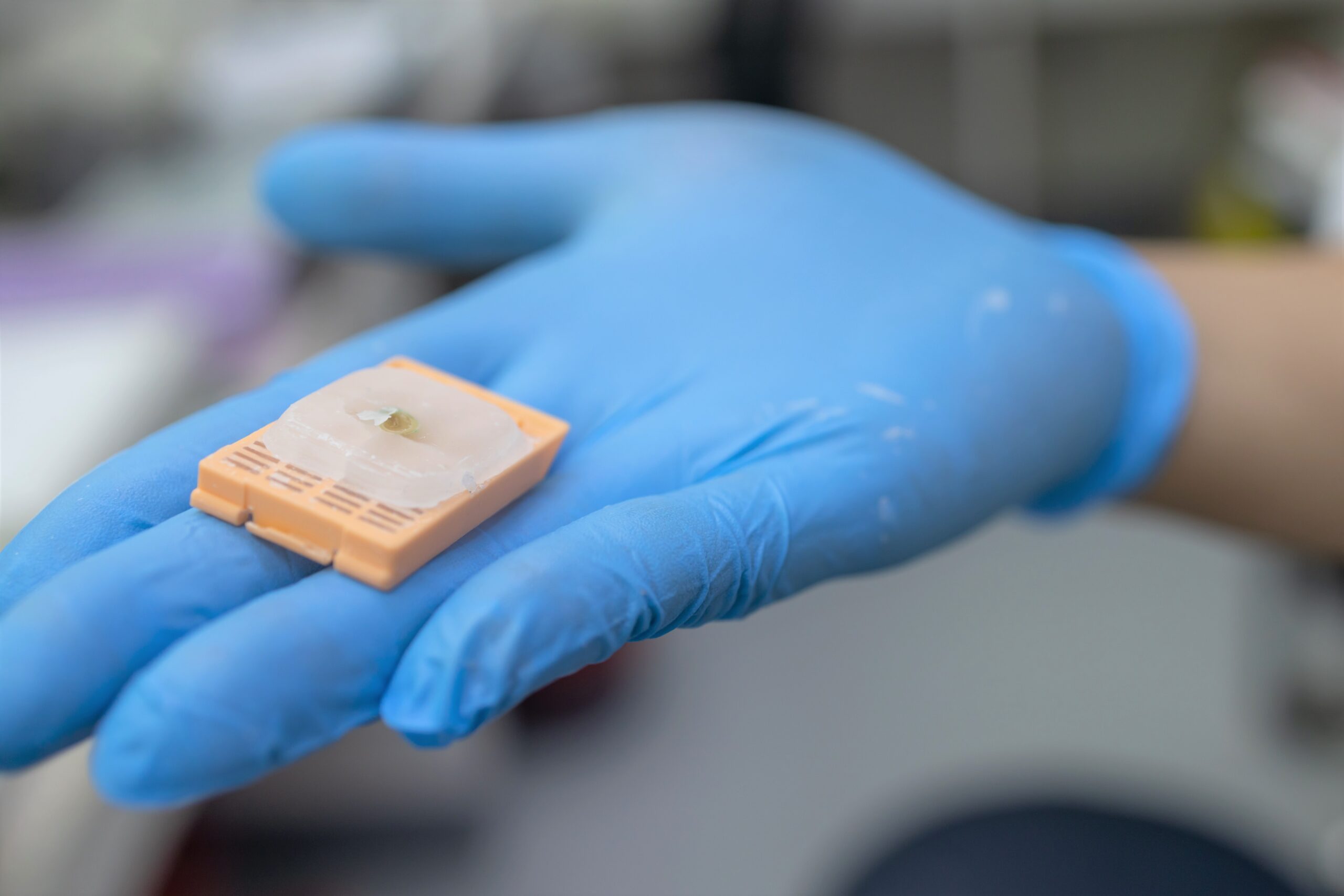
With the modern rise of next-generation sequencing (NGS) platforms, scientists are now looking to apply these powerful molecular technologies to the vast pool of existing FFPE samples. The following are a handful of key ways in which NGS technologies can be applied to FFPE tissue:
- Analysis of Clinical Samples: FFPE tissue samples are routinely collected in clinical settings and are often associated with extensive clinical and pathological data. By accessing the genetic material of these samples, researchers can perform retrospective studies to identify genomic alterations, discover and validate biomarkers, and pinpoint potential therapeutic targets. This allows for a better understanding of diseases, prognosis, and treatment options.
- Longitudinal Studies: FFPE samples are frequently collected over long periods of time, allowing for the analysis of samples from different time points within the same patient. This longitudinal analysis can provide insights into disease progression, treatment response, and the evolution of genomic alterations over time.
- Historical Archives: FFPE samples often come from archival collections of patient specimens, some of which may date back several decades. Accessing the genetic material in these samples enables retrospective studies that can shed light on the genetic basis of diseases and their historical trends. This can be particularly valuable in studying diseases with long latency periods.
Unfortunately, the fixation process also leads to extracted nucleic acids with poor quality, degradation, fragmentation, and deamination of cytosine bases, complicating transcriptomic-level investigations.2 “Formalin tends to crosslink the tissue to such an extent that it is hard to get fragments of DNA that are longer than, maybe, a hundred or two hundred base pairs,” says David Rimm, a pathologist at Yale University, who was interviewed by Nature in 2007 on this topic.1 The race is now on to generate methods for analyzing the degraded and modified biomolecules obtained from FFPE samples for molecular analysis.
Opening the Genetic Time Capsule
Historically, it has been prohibitively difficult or impossible to isolate quality nucleic acids from FFPE tissue suitable for downstream analysis, but more recent advances in DNA and RNA purification have enabled researchers to gather crucial genetic and molecular information from these samples. Common extraction techniques require de-waxing, proteolytic digestion, and purification before genetic-molecular analysis (see Figure 1).3
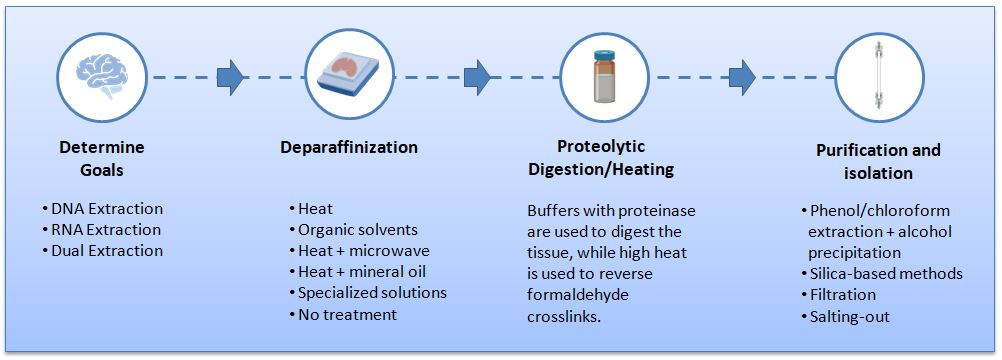
Paraffin removal is often done with xylene or other organic solvents, but there are alternate methods such as direct melting with microwaves, using mineral oil and incubation at 90°C, or using specialized extraction reagents.3 Most researchers agree that the de-waxing step does not have significant effects on downstream nucleic acid analysis, though the toxicity of the deparaffinization reagent is worth considering.3
The extraction of nucleic acids from FFPE tissues is the step that most impacts sample quality, because the reagents, incubation time, and incubation temperature involved in the extraction process have all been shown to significantly affect the quality of the DNA or RNA. Traditionally, researchers relied on high temperatures to remove crosslinks and adducts, but this is only partially effective and leads to additional fragmentation of labile nucleic acids such as RNA and denaturation of double-stranded DNA. Recently, new chemistry-based techniques for the reversal of crosslinking have been developed. The RNAstorm™ 2.0 FFPE RNA Extraction Kit and the DNAstorm™ 2.0 FFPE DNA Extraction Kit utilize proprietary CAT5™ catalytic technology to reverse crosslinking (Figure 2). Chemical de-crosslinking greatly accelerates the removal of formaldehyde damage and allows the use of milder conditions, resulting in markedly improved recovery of amplifiable nucleic acids.4
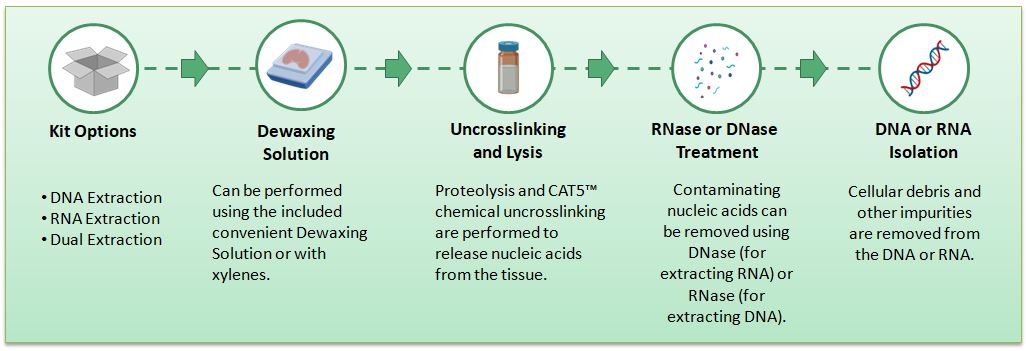
Compared to other methods, these kits greatly enhance the recovery of amplifiable nucleic acids suitable for a variety of applications, including NGS, qPCR, microarray, or other gene expression analysis.
Decoding the Genomic Cache with NGS
Sanger sequencing, the first method for sequencing DNA, was first described in 1977 and involved sequencing a single base at a time. A little under 30 years later, modern NGS was developed and greatly reduced the time and cost of genome sequencing. This second technological leap was based on parallel sequencing of millions of relatively short DNA fragments and required reconstruction of the short reads when sequencing long stretches of DNA. Common sequencing platforms from manufacturers like Ion Torrent, Illumina, and GeneReader utilize this technology. While revolutionary, second-generation sequencing can be challenging, especially in the presence of structural variations and low-complexity regions. This was the impetus for third-generation sequencers (e.g., Pacific Biosciences and Oxford Nanopore Technology instruments), which can read lengths of more than 10 kb and are able to directly target unfragmented DNA molecules in real-time. Unfortunately, due to the fragmented and relatively poor quality of nucleic acids extracted from FFPE samples, long-read sequencing platforms are typically not compatible with data gleaned from FFPE tissue.5 Therefore, there remains the need for more effective methods for extracting genetic material extraction from FFPE tissue as well as downstream sequencing.
From Tissue Banks to Genomic Discoveries
Optimizing the use of FFPE tissue with NGS promises great rewards in the form of access to a vast wealth of clinical and non-clinical data which can be used to study cellular processes in tissue. While challenges remain in extracting high-quality nucleic acids from FFPE tissue, recent advances in extraction methods offer hope for successful NGS analysis. Unlocking the genomic cache stored in FFPE tissue has the potential to revolutionize our understanding of diseases and pave the way for personalized medicine and scientific breakthroughs.
References
1. Blow, N. “Tissue Issues.” Nature vol. 448, no. 7156, 1 Aug. 2007. doi: 10.1038/448959a.
2. Tirado-Lee, L. “When Spatial Gene Expression Meets FFPE Tissue Blocks: A Modern-Day Love Story.” 10x Genomics, www.10xgenomics.com/blog/when-spatial-gene-expression-meets-ffpe-tissue-blocks-a-modern-day-love-story.
3. Bonin S., Stanta G. “Nucleic acid extraction methods from fixed and paraffin-embedded tissues in cancer diagnostics.” Expert Review of Molecular Diagnostics vol. 13,3 (2013): 271-82. doi: 10.1586/erm.13.14
4. Manjunath HS et al. “Gene Expression Profiling of FFPE Samples: A Titration Test.” Technology in Cancer Research & Treatment vol. 21 (2022): 15330338221129710. doi:10.1177/15330338221129710
5. Cappello, F et al. “FFPE-Based NGS Approaches into Clinical Practice: The Limits of Glory from a Pathologist Viewpoint.” Journal of personalized medicine vol. 12,5 750. 5 May. 2022, doi:10.3390/jpm12050750