Considerations for Doing RNA Work
Compared to working with DNA, working with RNA can be challenging and intimidating. While DNA is known to be quite stable and hardy (geneticists have been sequencing genomes that are thousands of years old), the chemical structure of RNA makes it prone to hydrolysis, making it much less stable. Both DNA and RNA can be degraded by nucleases, but RNase enzymes are highly stable and readily refold after denaturation, making them difficult to inactivate permanently. As part of the body’s viral defense system, they are secreted in skin, hair, and saliva, and so can readily contaminate experimental samples and cause problems for researchers. And finally, while there are many fluorescence-based reagents to quantify and visualize DNA, few are optimal for RNA, due to low sensitivity or a lack of specificity in the presence of contaminating DNA.
In this Tech Tip, we will simplify and demystify RNA work, and recommend tools that will help you to confidently isolate and analyze your RNA samples with ease.
RNA Handling
Good RNA hygiene starts with PPE (personal protective equipment). When working with RNA, it is important to always be aware of what you are touching with your gloves. Avoid touching your face or hair since that could allow transfer of RNase from your skin to your gloves. Change gloves if you have touched unclean equipment or surfaces. We particularly recommend always putting on fresh gloves before handing pure RNA samples.
Decontamination of surfaces and tools
Before beginning RNA work, it is a good idea to decontaminate your workspace, including the benchtop, your pipettes, and other equipment that you will be using. We recommend cleaning these surfaces by spraying them with RNase-X™ Decontamination Solution, wiping them down, then spraying them with either dH2O or 70% ethanol and wiping again. RNase-X™ is very efficient at removing RNases from surfaces (Fig. 1).
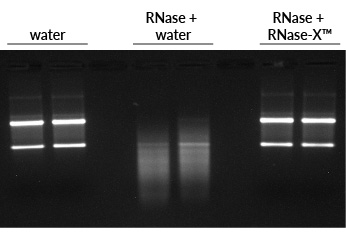
Tubes, tips, water, buffers, and reagents that will be used for RNA work must be RNase-free. Certified RNase-free products (such as Water, Ultrapure Molecular Biology Grade) can be purchased from many companies. Homemade solutions made with high grade ingredients and ultrapure water are often RNase-free; this can be verified using RNase testing assays such as RNaseAlert® (see RNase testing section below). Another common method is to treat solutions with DEPC (diethyl pyrocarbonate) to inactivate RNases. However, DEPC treatment has several drawbacks: it can’t be used with Tris-containing or other amino-containing solutions, it requires autoclaving, it may not fully inactivate RNases, it leaves the solution slightly acidic, and the DEPC by-products may inhibit downstream processes. So, we prefer to skip the DEPC, and use solutions that have been verified as RNase-free.
Apart from enzymatic degradation caused by RNases, RNA is also susceptible to hydrolysis, which is an intramolecular reaction that causes breakage of the phosphodiester backbone. EDTA has a protective effect because divalent cations like Mg2+ increase hydrolysis. RNA hydrolysis is worse in alkaline solutions, so RNA should not be stored in a solution with pH higher than 7.5. We have seen good stability of RNA stored in either citrate buffer pH 6 or TE buffer pH 7.5 (Fig. 2). Temperature is the most important factor in RNA stability during storage: for best stability and for long-term storage, RNA should be kept at -70°C. For short-term storage, we have found RNA to be stable at 4°C or -20°C for at least 3 weeks (Fig. 2).
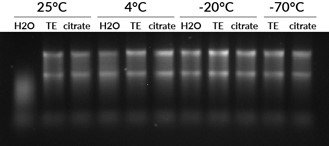
Some companies sell products like RNAlater™ that can be added to tissues or cells to keep the RNA stabilized during handling or storage. These stabilizers can offer flexibility to a researcher so that samples can be handled at room temperature, shipped, or treated to multiple freeze-thaw cycles without the RNA becoming degraded. However, the stabilization reagent must be removed, and the RNA purified, before any downstream work is done.
Another type of product is RNase inhibitor proteins, such as RiboGuard™ and others. These are proteins that inhibit specific types of RNases by binding to them in a 1:1 ratio. Their effectiveness depends on the amount of RNase contamination in a sample: trace amounts of RNase may be effectively inhibited, but samples containing larger amounts of RNase, for example RNase-treated DNA samples, may not show full RNase inhibition. Some caveats to using these RNase inhibitors are that they can be expensive, and their effects are non-covalent and reversible. Also, they contain glycerol and DTT, which may affect downstream applications. After attempting unsuccessfully to use RNase inhibitors to inactivate RNases in RNase-treated DNA, Biotium developed a proprietary method to permanently inactivate RNases in a solution, which we use to make RNase-Free Calf Thymus DNA.
When preparing solutions to use for RNA work (see Materials section above), it is useful to verify that the solution is free of RNase. Several companies sell RNase testing kits that use a fluorescently-labeled RNA oligo to measure RNase activity, for example RNaseAlert® from IDT. We have found these assays to be a good way to validate that homemade reagents and buffers are RNase-free. However, be aware that samples with extreme pH, high salt, or detergent can inhibit fluorescence-based RNase assays by directly affecting the fluorogenic RNase substrate or the RNase enzyme. This is easy to test for by adding RNase to your solution and ensuring that it registers fluorescence in the assay comparable to water plus RNase.
RNA Isolation
Isolation from fresh cells or tissues
For cultured cells and fresh tissues, total cellular RNA can be isolated by several different methods. The original method of extracting nucleic acids uses phenol and chloroform to separate proteins and lipids into an organic phase and nucleic acids into an aqueous phase. This process can be done under acidic conditions to selectively extract RNA away from DNA. A related method is to use TRI Reagent™ (also called TRIzol™), which uses guanidinium salt in combination with phenol and chloroform to selectively isolate RNA.
These days, many researchers prefer to use column-based RNA isolation kits to avoid the use of phenol-based methods that involve hazardous chemicals and tricky technique required to separate organic and aqueous phases. For those looking for a simpler purification solution, there are easy-to-use kits available such as the CELLDATA RNAstorm™ Fresh Cell and Tissue RNA Isolation Kit. Many such kits use guanidinium salt and silica membrane columns to selectively isolate RNA, while others use magnetic beads.
Total RNA isolated from these methods consists of ~95% ribosomal RNA. For some applications, it may be desirable to purify mRNA from the total RNA. However, because mRNA makes up a small percentage of cellular RNA, it can be difficult to obtain high yields of mRNA, and to remove all of the ribosomal RNA. Methods involving beads coated with oligo dT that bind to the poly A tails of mRNA are most commonly used to enrich mRNA from a sample.
Isolation from FFPE tissue sections
With the advent of next-generation sequencing methods, researchers increasingly wish to isolate RNA from formalin-fixed paraffin-embedded (FFPE) tissues, since patient samples are often preserved in this way. Purifying high quality RNA from FFPE tissues is more challenging than from fresh cells because formaldehyde chemically crosslinks the RNA to proteins and to itself. Some methods to reverse this crosslinking, such as high heat, can result in fragmented or poor-quality RNA.
The CELLDATA RNAstorm™ FFPE RNA Extraction Kit uses proprietary technology to enzymatically reverse formaldehyde cross-linking, avoiding the use of high temperatures and hazardous solvents. This leads to RNA with less fragmentation and greater amplifiability compared to other FFPE isolation kits, as measured by RIN score or by DV200 (Fig. 3).
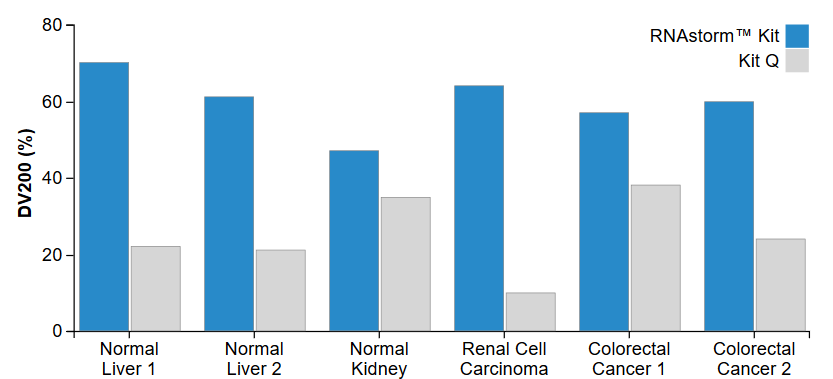
RNA Detection
The classic method of quantifying nucleic acids is by measuring UV absorbance at 260 nm (A260). Although A260 nm will not distinguish between RNA and DNA, it can be a useful way to quantify your RNA if you are certain that it is very pure (i.e., that there are no free nucleotides or DNA in your sample). For RNA, multiplying the A260 nm by 40 gives the concentration in ng/uL. It is typical to also take the absorbance at 280 nm to look for contaminants like protein that absorb strongly at 280 nm; a 260/280 ratio of 2.0 or higher is generally considered to be pure RNA. However, be aware that the 260/280 ratio can vary depending on pH. We recommend always using the same solvent, if possible, to make your absorbance readings consistent between experiments (we always use TE pH 7.5 to control pH for consistency).
Fluorescent dye-based RNA quantitation assays are the most accurate way to quantify RNA, because they have much greater sensitivity and linearity than A260 measurement. In addition, some (but not all) assays also have the advantage of being selective for ssRNA over dsDNA, so that you can achieve an accurate RNA concentration even in the presence of contaminating DNA. The AccuBlue® Broad Range RNA Quantitation Kit is highly selective for RNA over dsDNA (Fig. 4) and is accurate over a wide range of RNA concentrations. Be aware that some other companies’ fluorescent RNA detection dyes, such as RiboGreen®, while sensitive, are not RNA-specific, and will also detect dsDNA present in the sample.
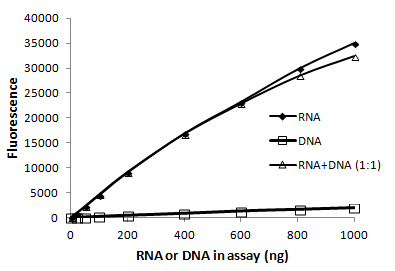
You may want to perform gel electrophoresis to determine the integrity or size of your RNA. It is often assumed that RNA electrophoresis always requires toxic, complicated formaldehyde gels to denature the RNA so that secondary structure doesn’t affect the migration through the gel. However, we find that is not necessary for evaluating RNA integrity. If your aim is just to visually evaluate your RNA, a regular agarose gel can be used, as long as a denaturation agent is included in the loading buffer. Adding EMBER500™ RNA Prestain Loading Dye to your RNA sample allows you to denature, load, and stain the RNA in a single step. The EMBER500™ dye is far more sensitive than ethidium bromide, so you can detect as little as 25 ng of total RNA (Fig. 5), and preserve your precious samples. RNA gel electrophoresis can also be done using polyacrylamide (PAGE) gels if you are looking at miRNA or other RNA up to 200 nucleotides long.
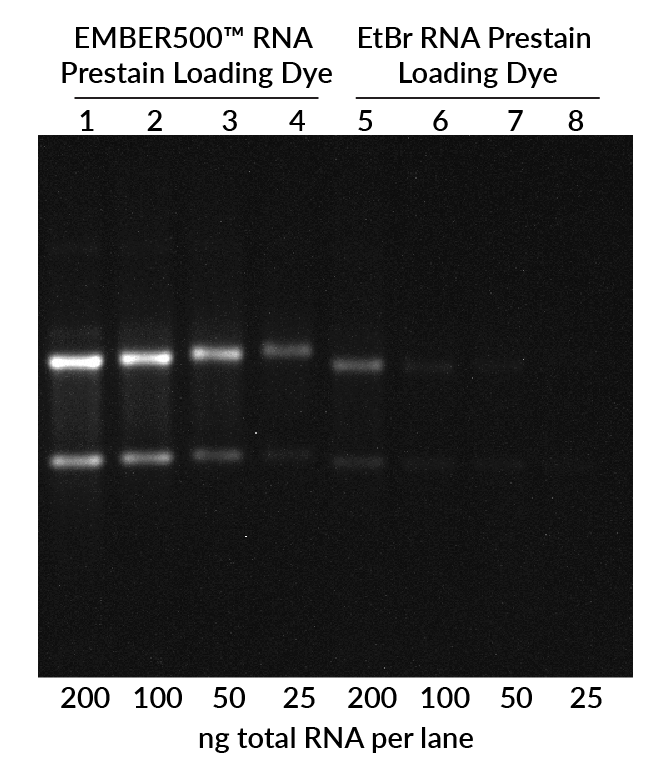