Greetings from the Biotium blog team! In this post we would like to present one of our guest writers, Dr. Christopher Pratt, who will talk about a powerful super-resolution imaging technique known as expansion microscopy. Dr. Pratt earned his PhD in cell biology and neuroscience from Carnegie Mellon University where he studied synaptic vesicle and ion channel trafficking in the brain using novel fluorogenic probes. Take it away, Dr. Pratt!
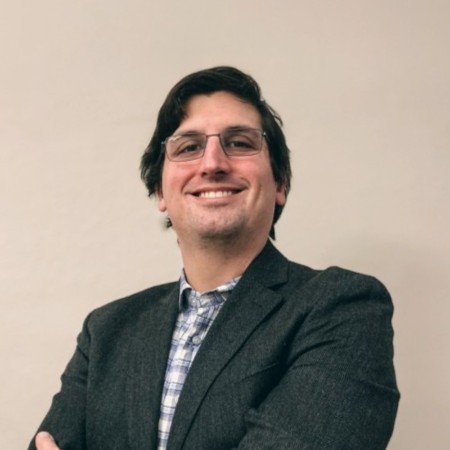
I met Dr. Ed Boyden in 2015 when he was awarded the Carnegie Prize in Mind and Brain Sciences at my graduate school. I was waist-deep in my thesis work developing novel fluorescent tools for microscopy, so having the chance to talk to an established innovator was thrilling. Ed Boyden’s claim to fame was his work developing optogenetics with Karl Deisseroth, but he told me about a new technique his group had just published where instead of breaking the diffraction limit to image smaller and smaller objects, they could enlarge the sample instead (Chen et al., 2015). Several years later, I experimented with expansion microscopy in my postdoctoral lab and learned a great deal about its limitations and its strengths.
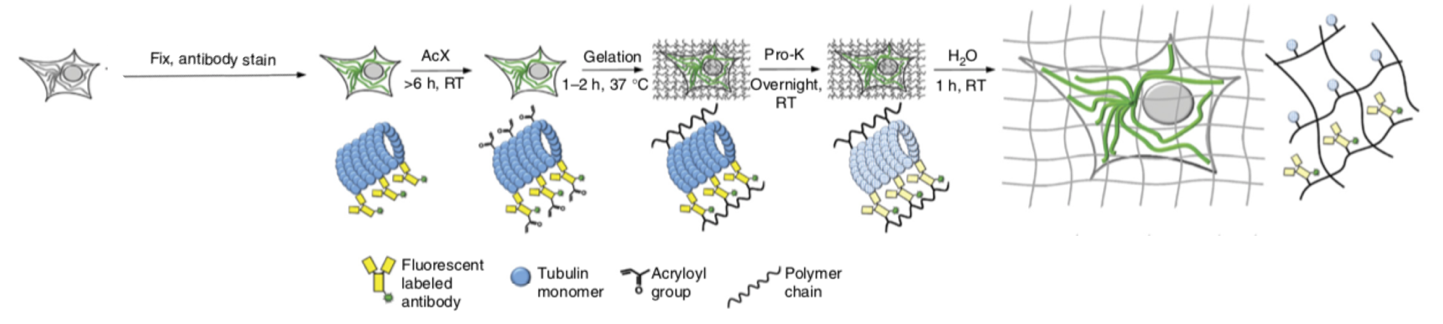
What is Expansion Microscopy?
In expansion microscopy, the fixed sample, either tissue or cells in culture, is embedded in a hydrogel matrix to which proteins are anchored. After the gel sets, water causes the gel to swell and expand isotropically, meaning that the spatial relationships between molecules are preserved in all 3 dimensions, like zooming in a picture. The reagents for making the gel and anchoring proteins are inexpensive, and the 4.5-fold sample enlargement offered by standard expansion microscopy brings the effective resolution to 50-100 nm, a significant improvement compared to the 200 nm resolution obtained from traditional light microscopy. This is an approach that is accessible to everyone for structural studies and doesn’t require expensive super resolution microscopes. Especially useful in tissue, expansion has the added effect of clarifying the tissue for deep imaging.
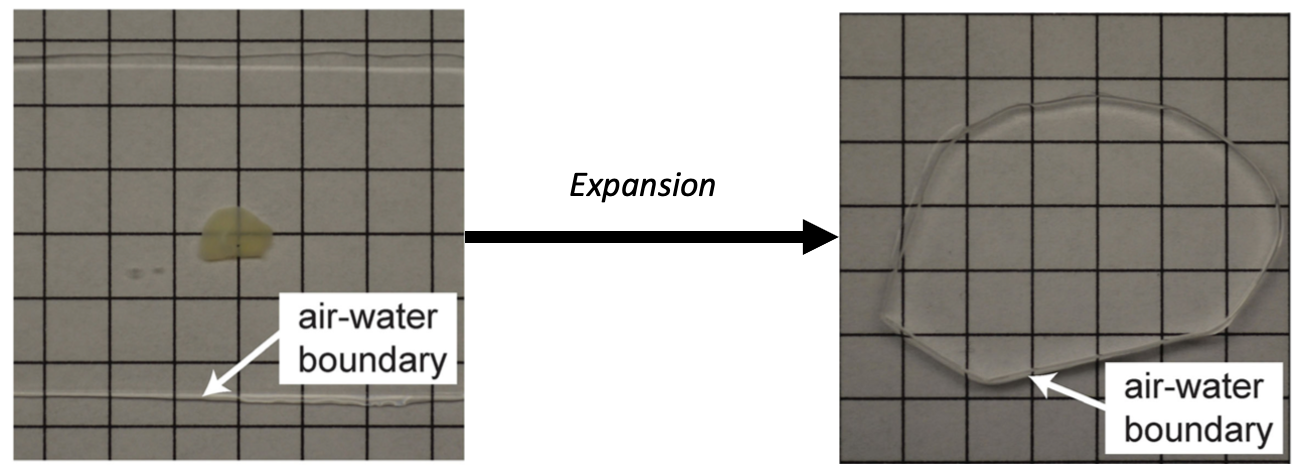
What Do You Need for Expansion Microscopy?
The reagents to create the hydrogel and anchoring solutions are common and inexpensive, and the protocols for anchoring, gelation, digestion, and expansion are fairly straightforward. Where I and many users find the most difficulty is in handling the expanded gel and mounting the samples for imaging. Fortunately, over the years, more and more publications with practical advice have appeared (R. Gao et al., 2017; Zhang et al., 2020), which will be immensely useful to new users.
There are considerations for expansion microscopy beyond the physical sample preparation. The most straightforward expansion microscopy technique involves performing immunostaining or expressing fluorescent proteins before gelation and expansion (Tillberg et al., 2016). Because the expansion dilutes the fluorophores by physically moving them apart, using bright dyes that survive treatment and are capable of fluorescing in pure water is crucial. Even before expansion, a 50% reduction in fluorescence intensity occurs from the anchoring and gelation steps (Tillberg et al., 2016). Several CF® dyes across the spectrum demonstrate excellent performance with expansion microscopy, including CF®405S, CF®405M, CF®488A, CF®568, CF®633, CF®640R, and CF®660R (Chen et al., 2015; Min et al., 2020; Tillberg et al., 2016).
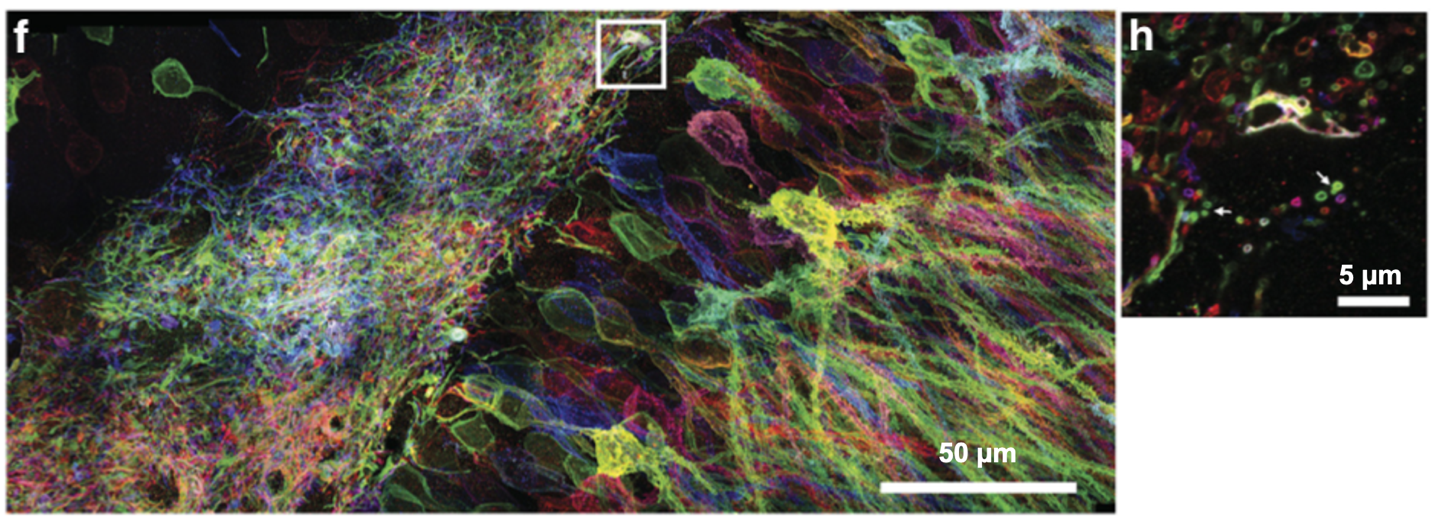
Developments and Trends in Expansion Microscopy?
Expansion microscopy continues to develop and work in combination with other techniques. The basic expansion technique allows about a 4.5-fold enlargement of a sample (Chen et al., 2015; Tillberg et al., 2016), but more involved methods like X10-expansion and iterative expansion microscopy increase the enlargement to 10 and 20-fold (Chang et al., 2017; Truckenbrodt et al., 2018), reaching an effective resolution of 25 nm on conventional microscopes. Expansion microscopy is an attractive option for super resolution imaging without the expensive hardware, but it has also been used with STORM, SIM, and STED to give unparalleled optical nanoscopic detail (M. Gao et al., 2018; Wang et al., 2018; Xu et al., 2019). Recently, ultrastructure-expansion microscopy has rivaled STORM in resolving the molecular organization of protein assemblies like the Chlamydomonas centriole and revealing molecular organization in mammalian cells (Gambarotto et al., 2019). With bright and reliable dyes, this technique can propel the understanding of molecular organization at even smaller scales than light microscopy alone will allow.
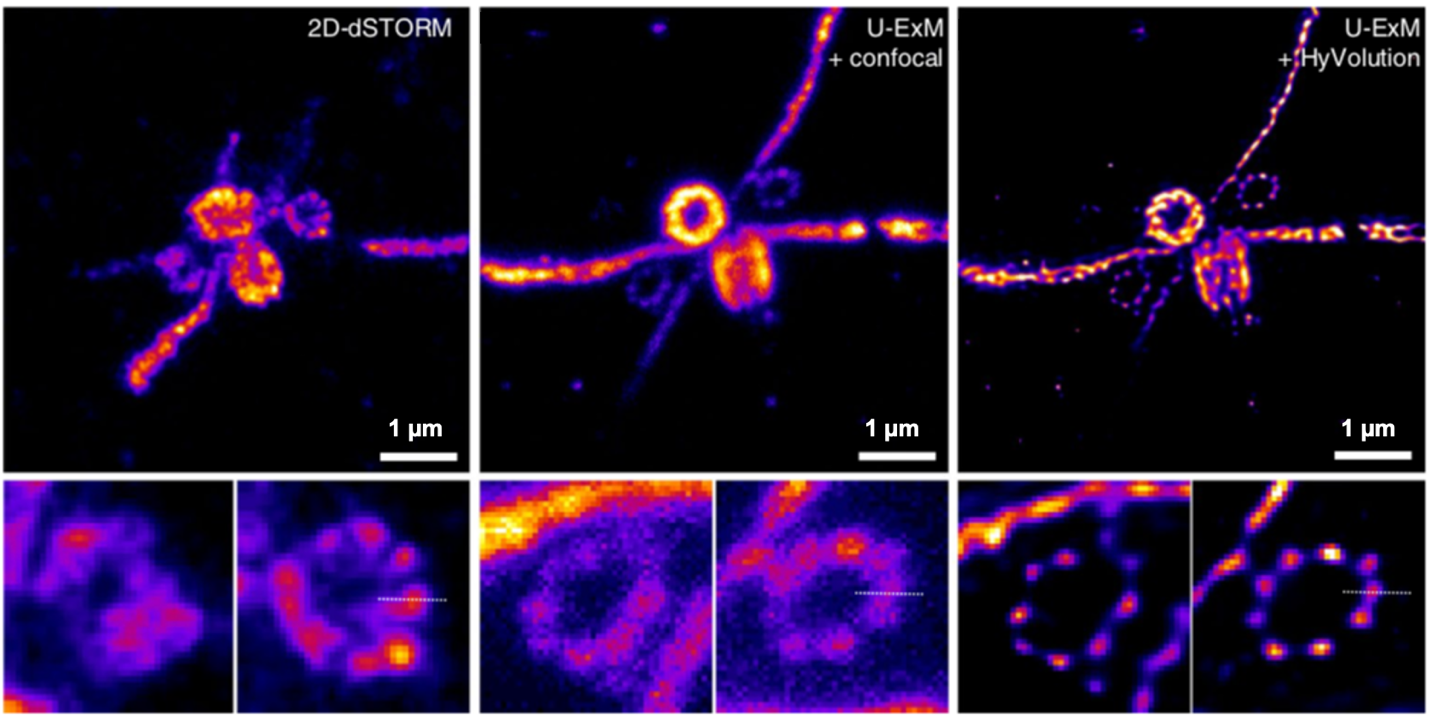
References
- Chang, J.-B., Chen, F., Yoon, Y.-G., Jung, E. E., Babcock, H., Kang, J. S., Asano, S., Suk, H.-J., Pak, N., Tillberg, P. W., Wassie, A. T., Cai, D., & Boyden, E. S. (2017). Iterative expansion microscopy. Nature Methods, 14(6), 593–599. https://doi.org/10.1038/nmeth.4261
- Chen, F., Tillberg, P. W., & Boyden, E. S. (2015). Expansion Microscopy. Science, 347(6221), 543–548. https://doi.org/10.1126/science.1260088
- Gambarotto, D., Zwettler, F. U., Le Guennec, M., Schmidt-Cernohorska, M., Fortun, D., Borgers, S., Heine, J., Schloetel, J.-G., Reuss, M., Unser, M., Boyden, E. S., Sauer, M., Hamel, V., & Guichard, P. (2019). Imaging cellular ultrastructures using expansion microscopy (U-ExM). Nature Methods, 16(1), 71–74. https://doi.org/10.1038/s41592-018-0238-1
- Gao, M., Maraspini, R., Beutel, O., Zehtabian, A., Eickholt, B., Honigmann, A., & Ewers, H. (2018). Expansion Stimulated Emission Depletion Microscopy (ExSTED). ACS Nano, 12(5), 4178–4185. https://doi.org/10.1021/acsnano.8b00776
- Gao, R., Asano, S. M., & Boyden, E. S. (2017). Q&A: Expansion microscopy. BMC Biology, 15(1). https://doi.org/10.1186/s12915-017-0393-3
- Min, K., Cho, I., Choi, M., & Chang, J.-B. (2020). Multiplexed expansion microscopy of the brain through fluorophore screening. Methods, 174, 3–10. https://doi.org/10.1016/j.ymeth.2019.07.017
- Tillberg, P. W., Chen, F., Piatkevich, K. D., Zhao, Y., Yu, C.-C. (Jay), English, B. P., Gao, L., Martorell, A., Suk, H.-J., Yoshida, F., DeGennaro, E. M., Roossien, D. H., Gong, G., Seneviratne, U., Tannenbaum, S. R., Desimone, R., Cai, D., & Boyden, E. S. (2016). Protein-retention expansion microscopy of cells and tissues labeled using standard fluorescent proteins and antibodies. Nature Biotechnology, 34(9), 987–992. https://doi.org/10.1038/nbt.3625
- Truckenbrodt, S., Maidorn, M., Crzan, D., Wildhagen, H., Kabatas, S., & Rizzoli, S. O. (2018). X10 expansion microscopy enables 25‐nm resolution on conventional microscopes. EMBO Reports, e45836. https://doi.org/10.15252/embr.201845836
- Wang, Y., Yu, Z., Cahoon, C. K., Parmely, T., Thomas, N., Unruh, J. R., Slaughter, B. D., & Hawley, R. S. (2018). Combined expansion microscopy with structured illumination microscopy for analyzing protein complexes. Nature Protocols. https://doi.org/10.1038/s41596-018-0023-8
- Xu, H., Tong, Z., Ye, Q., Sun, T., Hong, Z., Zhang, L., Bortnick, A., Cho, S., Beuzer, P., Axelrod, J., Hu, Q., Wang, M., Evans, S. M., Murre, C., Lu, L.-F., Sun, S., Corbett, K. D., & Cang, H. (2019). Molecular organization of mammalian meiotic chromosome axis revealed by expansion STORM microscopy. Proceedings of the National Academy of Sciences, 116(37), 18423–18428. https://doi.org/10.1073/pnas.1902440116
- Zhang, C., Kang, J. S., Asano, S. M., Gao, R., & Boyden, E. S. (2020). Expansion Microscopy for Beginners: Visualizing Microtubules in Expanded Cultured HeLa Cells. Current Protocols in Neuroscience, 92(1), e96. https://doi.org/10.1002/cpns.96